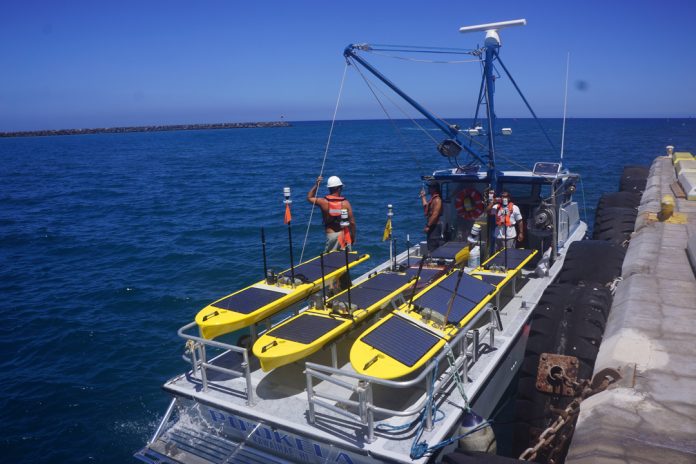
In the long history of human seafaring, our species has found ways of harnessing the energy from the environment and eking out meagre on-board stores to achieve increasingly amazing feats of endurance, sometimes with tragic costs.
For most of that time the vital seafaring technology was sail, oar and human muscle, not to mention the advent of the internal combustion engine and later nuclear power which also changed the face of seafaring. Nevertheless, today unmanned underwater and surface ‘gliders’ respectively harness changes in buoyancy and energy in waves to propel them for many months at low average speeds. This creates possibilities for a wide variety of missions that are beginning to come to fruition, and these different vehicle technologies are mutually supportive. Although both the buoyancy and wave energy propulsion technologies have been in use for years, they are still sufficiently unfamiliar to make a brief description of their operating principals worthwhile, before exploring how their missions and technology are developing.
Buoyancy for propulsion
All buoyancy-driven gliders become denser or less dense than the surrounding water by pumping a fluid, usually oil, between bladders inside and outside their pressure hull. The energy for the pumping action usually comes from a battery, but a water temperature gradient can provide it, more of which later. Changing buoyancy causes the vehicle to rise or fall within the water column, while the lift vector of the wings induces forward motion so that the vehicle progresses in a sawtooth pattern, moving slowly but economically; half a knot/kt (0.6 kilometres per hour) on half a Watt is the rule of thumb. Although some feature hydrodynamic control surfaces, steering is often accomplished simply by moving the weighty battery pack from side to side to induce a roll, and fore and aft to induce a pitch change.

Payload packages typically contain Conductivity, Temperature and Depth (CTD) sensors whose output feeds into Sound Speed Profiles (SSPs) which are graphs that plot local sound speed against depth. These provide vital information for sonar performance calculations used by other Anti-Submarine Warfare (ASW) and mine countermeasures platforms. Buoyancy driven underwater gliders are generally limited to fairly low-data-rate communications systems, including acoustic modems and satellite communications (SATCOM) links. At regular intervals they come close to the surface to expose a SATCOM antenna so they can download their mission and housekeeping data and receive new instructions.

Power from waves
Wave-driven Unmanned Surface Vehicles (USVs) such as the Boeing/Liquid Robotics’ Wave Glider, for example, use pivoting foils to harness wave energy to propel them in any direction, regardless of the direction from which the waves are coming. The Wave Glider exploits vertical ‘heave’ motions to drive forward motion using foils attached to a module tethered underneath the floating hull by a cable long enough to put it in a body of water that is not subject to the wave motion that moves the hull up and down. This forces the foils vertically through this relatively stable water column so that they generate thrust by pivoting to the angle of least resistance. Moreover, the Wave Glider can use a combination thruster and rudder called a Thrudder, which provides steer-able auxiliary thrust when waves are very small, as in the doldrums, or when currents are very strong. When needed, the Thrudder adds about 0.5kt to the Wave Glider’s speed.

Buoyancy Glider Genesis
With funding from the US Office of Naval Research, development of buoyancy-driven gliders began in the early 1990s, noted Gerald D’Spain from the University of California in San Diego (UCSD). He added that these vehicles, such as the Spray glider from Scripps Institution of Oceanography (SIO), the Seaglider from the Applied Physics Laboratory at the University of Washington, and the Slocum glider originally developed at the Woods Hole Oceanographic Institution, can be hand-deployed by two people. Over the last decade or so, they have come into much more routine use in data collection for US federal and state resource management agencies such as the National Oceanographic and Atmospheric Administration and for the oil and gas industry, he pointed out. They have also been equipped with a wider variety of small, low-power sensors and with small computers running autonomous algorithms that can make independent decisions based on information collected by on-board sensors, he added.

While other missions beckon, the principal naval use of buoyancy-driven gliders remains oceanography, which over the last decade or so have benefited greatly from advances in worldwide command and control, improvements in real-time data transfer, additional sensors and extended mission life, according to a spokesperson for the US Navy’s Naval Meteorology and Oceanography Command (NMOC). The US Navy owns more than 100 gliders, the world’s largest fleets, most of them produced by Teledyne Marine.
Beyond monitoring of ocean with CTD sensors to support sonar operations, US Navy Oceanography commands use glider technology for environmental data collection for improvements to models in order to better understand the oceans and support naval operations, the spokesperson said: “We’re open to exploring any innovative use of gliders or attached sensors that can help enhance the mission.” Gliders have to be very energy efficient to remain effective at sea for long periods, both in terms of propulsion and payload systems: “The current sensor payload uses very little power. The movement of the displacement system for changing the buoyancy is the major power consumer in a glider and limits the number of dives the vehicle is able to accomplish in a single sortie”, the spokesperson continued: “The sensor systems for the Slocum gliders we utilise in Naval Oceanography are very efficient. This high efficiency in power management enables resolution of sampled features over time and space at a substantially lower cost than with traditional shipboard methods.” Mr. D’Spain emphasised that gliders’ frugal use of energy is primarily a function of their slowness. He told Armada that for any mobile platform, the rate of propulsion energy consumed per unit time increases with the cube of the speed with respect to the medium through which it travels. In other words, doubling of the speed of an underwater vehicle causes an increase in power consumption by a factor of eight: “Underwater glider propulsion is so energy efficient with respect to energy consumed per unit time because gliders move so slowly through the medium.”

A more relevant measure of propulsion efficiency is energy consumed per unit of distance travelled, he said, to which the Lift-to-Drag (L/D) ratio is directly related: “The flying wing concept maximizes L/D, so that gliders based on it consume less energy per horizontal distance travelled than any other comparably-sized glider travelling at a comparable speed.” A new type of glider that exploits these principles has been deployed at sea Mr. D’Spain told Armada. Larger and faster than earlier types, the flying wing glider and is optimised for long distance, long duration missions: “Their significantly larger size (6.1 metre/20 feet wingspan) also permits increases in speed, hydrodynamic efficiency, payload capacity and cargo-carrying capabilities.”
Energy Storage
As with other Autonomous Underwater Vehicles (AUVs), advances in energy storage and harvesting promise to increase their range and endurance and provide more energy for sensor payloads, although there is still work to do before they can be deployed operationally, stated Dan Rudnick, professor of climate, atmospheric science and physical oceanography, at UCSD, who developed the Spray glider: “Systems that use alternate sources of energy, like the ocean’s thermal gradient, and seawater batteries, are certainly being developed,” he said: “I would not classify the ones I know as mature.” Mr. D’Spain gave the example of phase changing paraffin waxes that have been used successfully to demonstrate buoyancy driven platforms’ ability to ‘feed’ off changes in temperature with depth in the ocean in some environments.
He recalled a partnership between Scripps and the National Aeronautics and Space Administration’s (NASA) Jet Propulsion Laboratory to demonstrate the operation of a buoyancy-driven profiling float that operated at sea for more than a year with a buoyancy change system powered by a thermal engine, the technology for which was developed by Doug Webb, the founder of Webb Research (now part of Teledyne Marine) when he worked at Woods Hole: “Maturity of this technology can be demonstrated by whether or not such a thermal-engine-equipped glider is available on the market.” An important point about phase-changing materials that extract energy from thermal gradients, such as paraffin waxes, is that they shrink in volume as they freeze and expand as they melt, which is the wrong direction for direct use as a buoyancy engine: “The engine must expand the glider’s volume when it gets to the bottom of its dive cycle and shrink its volume at the top”, he said: “Therefore, a thermal glider must have an energy storage system that allows the energy extracted from the phase change to be available at the next half-cycle. For example, this phase-change energy could be used to help recharge the onboard batteries.”
Prof. Rudnick explained that propulsion is usually allocated about 60 percent to 70 percent of the energy budget of UCSD’s gliders, depending on the sensors carried: “So sensors take up a smaller part of the energy budget (typically 20 percent to 30 percent), but they often determine endurance because they are what we more often change, while energy for propulsion remains constant.” The rest, roughly 10 percent, is accounted for by everything else including the gliders’ computer, communication and navigation. UCSD is exploring ways of packing more energy into gliders, including the simplest expedient of all: “The easiest way to increase energy capacity is to make gliders bigger, which we are considering. Other than that, better batteries would help,” Prof. Rudnick concludes.
Enabling ASW
Interest in gliders has always extended beyond scientific oceanography and, as Mr. D’Spain points out, their transition to operational navies has begun and he expects this market to continue to grow. In mid-November 2016, the service announced that it was ready to deploy gliders from its destroyers to provide an edge to fleet ASW capabilities following approval from US Navy’s Naval Sea Systems Command (NAVSEA) which cleared the deployment of up to two gliders each from ‘Arleigh Burke’ class destroyers after successful testing in the Pacific. The AUVs will provide destroyer sonar operators with real-time data. This will most likely come from CTD sensors and be used to update SSP diagrams for sonar calibration on surface and airborne platforms.
While their direct use for submarine detection with passive sonar sensors, for example, is an obvious possibility it is not one that the service is talking about openly. They are, however, discussing the detection, tracking and study of other underwater entities, as Mr. D’Spain explained: “The US Navy is interested in better understanding the distribution and behaviour of marine mammals, endangered species, and other marine life in order to help minimize the potential of naval activities to impact the ocean environment.” This is a mission for the new larger gliders with acoustic sensors, he explained: “The ‘flying wing’ autonomous underwater gliders are equipped with a multi-element hydrophone array installed all along the leading edge of the wing, as well as additional underwater acoustic sensors, in order to quietly listen to, and determine the direction towards, sounds in the ocean. Therefore, these gliders are well suited for detecting, localising, and tracking individual sound-producing animals in the ocean.”
Tracking an individual marine mammal with a single glider requires the animal to emit sounds sufficiently often that its sequence of calls can be associated with it, he said, and that the glider can determine the direction from which each call is coming in order to develop a track: “Whereas prop-driven propulsion systems create sound continuously, some species of marine mammals often don’t call sufficiently often for a track to be established. Other species occur in such tight groups and generate sounds so often (like pods of porpoises and dolphins) that tracking individuals within a pod is virtually impossible.” Mr. D’Spain emphasised that ‘flying wing’ gliders may be the only category with sufficient payload capacity to carry large aperture arrays on board. The alternative, he said, is to tow such an array, which can be challenging: “Tracking of populations of calling marine mammals can be accomplished by a spatially distributed set of gliders, where each glider listens to determine presence/absence of the particular species of interest.”
Missions
USVs that exploit wave energy, such as the Wave Glider boast even greater endurance than most underwater gliders because they can recharge their batteries from solar panels and because they run on the surface, can remain in constant communication and can continuously receive position updates from Global Positioning System satellites. Liquid Robotics calls the Wave Glider a transformational technology that can help build the “digital ocean” in that it is ideally placed to act as a communications gateway from the sea floor to space, enabling underwater vehicles including buoyancy-driven gliders equipped with acoustic modems to communicate globally without surfacing: “We are part of a larger ‘system of systems’ connecting inhabited and unmanned assets. This is a critical infrastructure needed to help unlock the 95 percent of the ocean that is currently unexplored and to assist in solving some of the world’s largest challenges,” the company told Armada.
Wave Gliders, said the company, have logged more than 1.1 million nautical miles (2.1 million kilometres) at sea. While the vehicles have been proving themselves, the company emphasised that it has been working on advances in power, energy capacity, sensor payloads, communications, durability and software with a strong focus on autonomy. Liquid Robotics says that Wave Gliders can stay at sea for periods of several months up to a year, depending on the mission, the limiting factors being bio-fouling or barnacle growth on the vehicle and its sensors, the sea state and the amount of solar energy available. These factors depend heavily on the season in which the glider is deployed, its location and types of sensors. Barnacle growth is heavy in the Gulf of Mexico in the summer, for example, affecting sensor operation to the extent that the gliders have to be cleaned, a problem that operators address with a relief system in which fresh gliders swim out and fouled ones swim home for a scrub.
Anti-submarine warfare, weather for situational awareness and long duration surveillance are the primary naval missions, which determine the core meteorological and acoustic sensor suites that equip them. Like other AUV developers, Liquid Robotics and Boeing participate in exercises and warfighting experiments such as the Unmanned Warrior event that took place in waters off the United Kingdom in the autumn of 2016, in which Wave Gliders demonstrated their ASW prowess and Geospatial Intelligence (GEOINT) gathering and dissemination credentials. The vehicles also took part in a set of GEOINT exercises led by the UK National Oceanographic Centre called Marine Autonomous Systems in Support of Marine Observations (MASSMO). In MASSMO, they gathered what the company described as valuable and consistent information about water temperature, currents and other details across multiple vehicles in difficult ocean conditions: “We also demonstrated how data from multiple Wave Gliders could be integrated into third party systems, such as those offered by Boeing, to facilitate operational decision making.”
Looking into the foreseeable future, the expectation in the NMOC is that further increases in endurance and sensor payloads should be a primary focus of development: “The information collected is such a benefit to the Navy and a major input to Navy ocean models. The future is strong for glider operations in the Navy”, the NMOC spokesperson said: “I think the most important directions will be to make gliders easier to use by non-experts,” Mr. Rudnick noted, “which is essential to getting more gliders into the water.” Buoyancy and wave driven autonomous vehicles represent a youthful technology with plenty of naval potential to be explored, technology that submariners will have to take very seriously.
